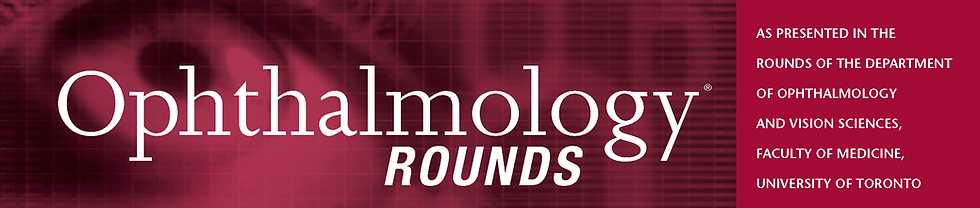
Looking Into Diabetic Retinopathy:
Features on Optical Coherence Tomography Angiography
By Paulo Ricardo Chaves de Oliveira, MD, Alan Richard Berger, MD, FRCS,
and David Robert Chow, MD, FRCS
With the worsening global pandemic of diabetes, millions more patients are at significant risk of diabetic retinopathy (DR). The development of optical coherence tomography angiography (OCTA) has provided a potentially valuable tool to detect and evaluate retinal disorders, including DR, while avoiding the adverse effects of the more traditional fluorescein angiography. This issue of Ophthalmology Rounds discusses the benefits and limitations of OCTA in assessing DR.
The global prevalence of adults with diabetes is currently 424.9 million, or 8.8% of the world’s adult population, and is expected to reach 628.6 million (9.9%) in 2045, due primarily to the rise of obesity and unhealthy lifestyles.[1] In Canada, 9.3% of the population (3.4 million) have diabetes, and the prevalence is projected to increase to 12.1% (5.0 million) by 2025.[2] Another 22.1% of Canadians aged ≥20 years are prediabetic (Table 1).[2,3] All of these patients will be at risk of developing diabetic retinopathy (DR), one of the leading causes of blindness worlwide.[4-6] The Meta-Analysis for Eye Disease (META-EYE) Study Group estimated that approximately one-third of diabetes patients have DR, and one-third of this group had vision-threatening DR.[4} The prevalence of DR was shown in some studies to be increasing steadily;[7,8] however, a 10-year analysis of participants (N=7.7 million), the United Kingdom Clinical Practice Research Datalink, revealed that the prevalence of DR remained stable among type 2 diabetes patients and declined in the type 1 diabetes subgroup.[9]
Table 1: Canadian Diabetes Association definition of prediabetes[3]

DR is characterized by different levels of capillary occlusion, vascular hyperpermeability, and neovascularization in the retinal vasculature.[10-12]
Fluorescein angiography (FA), first introduced in 1961 for the study of retinal vasculature, is an important and well established tool for the evaluation of retinal disorders, including the severity of DR. According to the pattern of dye distribution, microaneurysms can be identified, along with intraretinal microvascular abnormalities, macular edema, areas of ischemia/non-perfusion, and neovascularization of the disk or elsewhere. FA is a time-consuming, invasive procedure, however, and is associated with a risk of adverse reactions ranging from nausea to anaphylaxis.[13-17]
Optical Coherence Tomography Angiography (OCTA)
A relatively new technology, OCTA allows the study of the chorioretinal vasculature without the need for contrast agents. Different techniques may be employed to detect flow, such as measuring the changes (decorrelation) in the reflected OCT signal intensity (amplitude) between consecutive cross-sectional B scans taken at the exactly same position, or assessing changes in the phase of the reflected light waves under successive acquired B scans (phase variance). A combination of these 2 techniques is also possible. The OCT signal from static tissue will show little changes over time, while moving components, such as blood cells, will have significant variations. These variations are assumed to originate from flow, generating blood motion contrast and creating angioflow maps.[18-20] The angioflow maps are evaluated en face and automatically shown in 4 slabs: the superficial vascular plexus, the deep plexus, outer retina, and choriocapillaris (Figures 1A-1D).
Figures 1A-1D: 3 x 3 mm optical coherence tomography angiography (OCTA) in a 32-year-old
healthy patient using co-registered OCT B-scans (below each OCTA image). A. Superficial plexus. B. Deep plexus. C. Outer retina, which is normally avascular. D. Choriocapillaris.
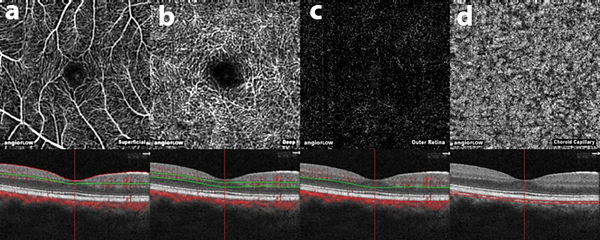
-
The superficial vascular plexus corresponds to the vessels normally seen in a routine FA examination, located in the ganglion cell layer and in the retinal nerve fibre layer
-
The deep plexus consists of 2 plexuses located on the inside of the inner nuclear layer and on the outside of the outer plexiform layer, and was not previously appreciated in FA examinations
-
The outer retina normally does not contain vessels
-
The choriocapillaris shows the superficial choroidal vasculature, below Bruch's membrane
The en face images can be scrolled anywhere to examine different areas of interest. The currently available fields of view are 2 x 2 mm, 3 x 3 mm, 6 x 6 mm, and 8 x 8 mm, although montage techniques are possible in order to cover wider angles. Since the number of scans is always the same, details are lost by moving from minimal to wider fields of view.[20]
OCTA in the Assessment of DR
Microaneurysms
Microaneurysms are generally detected as hyperfluorescent dots on FA, during the early phases of the examination. On OCTA, microaneurysms may present in a variety of shapes, such as focally dilated saccular, fusiform, or round with dark-centre capillaries, and may originate from the superficial or deep plexus (Figures 2A-2C).
Figures 2A-2C: OCTA in a 49-year-old patient with proliferative diabetic retinopathy (DR; neovessels not shown). A. Fluorescein angiography of the macular area with approximately 3 x 3 mm. Examples of microaneurysms (dotted circles) assessed by 3 x 3 mm OCTA images of the superficial (B) and deep (C) vascular plexuses. Nonflow areas and irregular foveal contour are also observed.
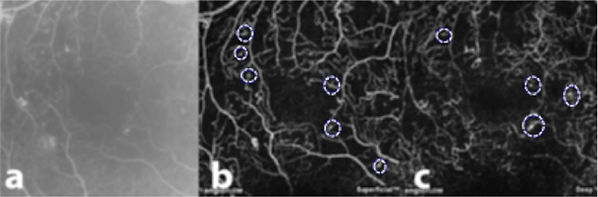
Previous studies have reported conflicting opinions regarding the value of FA and OCTA in the detection of microaneurysms.[12,21-23] Matsunaga et al described that they may appear only on FA, only on OCTA, or both.[21] In addition, it seems that more microaneurysms are evident on FA than on OCTA, which could be related to their histological features (consisting of diverse components and contributing to a turbulent flow) being below the slowest detectable flow by OCTA. Furthermore, some microaneurysms may be patent to the smaller fluorescein molecules (and therefore, detected on FA) but not to red blood cells, not having flow through them and consequently not being depicted on OCTA. On the other hand, some OCTA microaneurysms not appreciated on FA may, in fact, simply correspond to capillary ends or vertically oriented capillaries.[21-23]
Foveal avascular zone (FAZ)
Previous studies have reported alterations in the FAZ of diabetes patients, and its enlargement may be an indicator of DR progression (Figures 3A-3D).[24,25] OCTA is able to clearly show details of the FAZ without the concern of dye leakage.[26-28] As assessed by OCTA, DR patients may present with a larger FAZ area and disruption of the capillary network in both the superficial and deep vascular plexus when compared to healthy control subjects. Actually, it seems the FAZ changes may be more accentuated at the level of the deep plexus, not previously appreciated by FA.[26-28] The differences in the FAZ features were also demonstrable when comparing diabetes patients without DR and healthy subjects.[29] Therefore, OCTA seems to show alterations in the FAZ before the development of clinically detectable DR, potentially functioning as a screening tool. Future studies are needed to validate these findings.
Figures 3A-3D: Foveal avascular zone in DR. 3 x 3 mm OCTA of the superficial (A) and deep (B) vascular plexus of a 32-year-old healthy patient, showing regular contour of the foveal avascular zone (FAZ) and normal vessel distribution. 3 x 3 mm OCTA of a severe nonproliferative DR of the superficial (C) and deep (D) vascular plexus. Note the enlargement and disruption of the FAZ area (blue dashed lines). Non-flow areas (asterisks), vessel tortuosity, and loops are also appreciated.
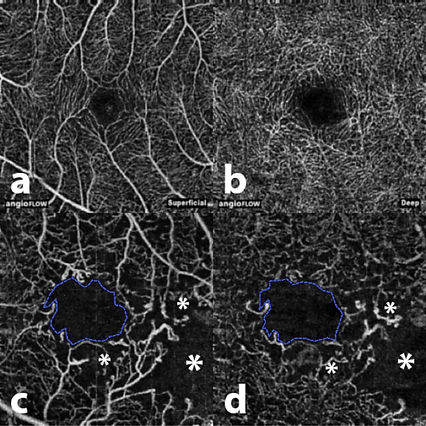
Capillary dropout / nonperfusion areas
Areas of capillary dropout / nonperfusion are depicted as dark spaces on OCTA, devoid of capillaries, showing good correlation with FA (Figures 4A, 4B). In some instances, OCTA is able to show additional areas of impaired perfusion since it is not obscured by diffuse dye leakage. In these cases, OCTA is more consistent than FA in demonstrating the presence or absence of retinal capillaries as well as of retinal abnormalities identified at the edges of nonperfusion areas.[12,21,22]
Figures 4A, 4B: Nonperfusion / capillary dropout assessed by OCTA. A. 6 x 6 mm OCTA of the superficial plexus of a 40-year-old healthy patient. B. 6 x 6 mm OCTA of an aged-matched subject with severe nonproliferative DR, showing nonperfusion areas (asterisks), irregular FAZ contour, vessel tortuosity and vascular loops.
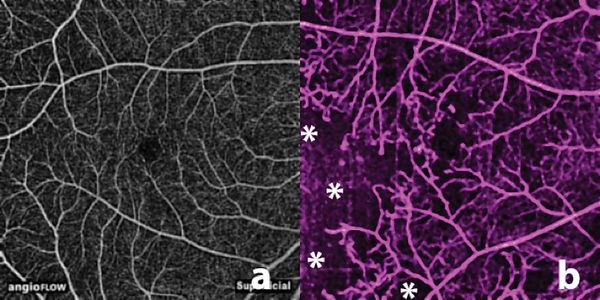
Neovascularization
Proliferative DR (PDR) is characterized by the presence of neovessels of the retina or disc (Figures 5A, 5B) that grow into the vitreous through a break of the internal limiting membrane (ILM).[30] Since OCTA is depth-resolved, different areas of interest can be visualized. By segmenting the angiograms to project blood flow from above the ILM in the vitreous cavity, OCTA can detect the existence of neovessels.[31-33] In their evaluation of PDR patients, de Carlo et al[31] were able to demonstrate the presence of preretinal neovascularization in 13 eyes assessed by OCTA. They also showed that neovascularization was noted to border an area of capillary nonperfusion in 92% of the cases, further supporting the theory that retinal hypoxia plays a role in the development of neovascularization.
Figures 5A, 5B: OCTA in a case of proliferative DR with neovessels of the disc (NVD; blue arrows). A. Colour retinography of the optic disc, showing the fundus neovascularization appearance. B. OCTA at the optic disc segmented with the inner boundary in the vitreous above the NVD and the outer boundary below the internal limiting membrane.
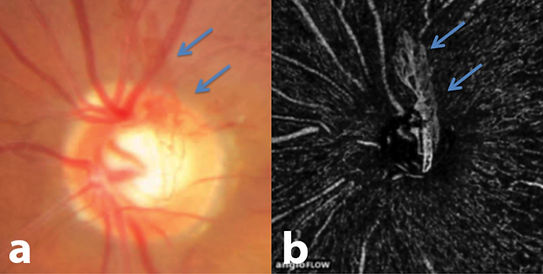
The morphology of the neovessels in PDR has also been assessed by OCTA.[32,33] Ishibazawa et al[32] described that neovessels in patients with PDR could be morphologically divided into vessels with and without exuberant vascular proliferation (EVP). Those with EVP are characterized by intense growth of irregular small-calibre vessels located at the margin of neovessels, which likely represents active proliferation. Almost all non-treatment patients in the study had EVP, while the rate of EVP in neovessels of patients previously treated with panretinal photocoagulation was much lower, some of them presenting only with filamentous vascular loops. The authors also stated that the concordance between the presence of EVP in OCT angiography and significant leakage in early-phase FA may indicate that the presence of EVP on OCTA should be interpreted as an active sign of new vessels in clinical practice.
Macular edema
Diabetic macular edema (DME) is the most common cause of vision loss in diabetes patients. OCTA may offer the advantage of noninvasive detection of cystoid changes and adjacent microvascular abnormalities (Figures 6A-6C). de Carlo et al[34] demonstrated that DME may be identified as rounded areas of black flow voids representing intraretinal cystoid spaces, and were more evident in the deep vascular plexus. The structural OCT B-scan and structural en face OCT can be evaluated simultaneously to assess the exact location of intraretinal fluid.[34,35]
Figures 6A-6C: A 54-year-old male patient with diabetic macular edema. A. OCTA image of the deep vascular plexus of the inner retina showing intraretinal cystoid spaces (blue arrows), observed as oblong black areas devoid of flow with smooth and rounded borders. B. En face structural OCT showing the cystoid spaces in the correspondent distribution. C. Co-registered OCT B-scan showing the deep vascular plexus OCTA segmentation in A (green lines).
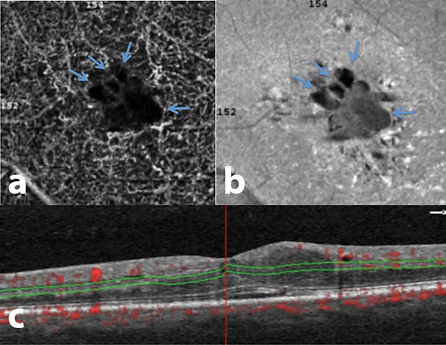
Newer Technology Developments
New device improvements allow the use of OCTA for quantitative analysis of chorioretinal disorders, including DR (Figures 7A-7D).[14,22,36] Some of the commercially available software incorporate the assessment of flow / nonflow areas and FAZ area, as well as the retinal vascular perfusion density values of the different vascular layers. Colour coded, vascular density maps are also automatically created: red represents a density of >50% perfused vessels, dark blue represents 0% perfused vessels, and intermediate perfusion density are colour coded accordingly.
Figures 7A-7D: OCTA quantitative analysis. Colour-coded vessel density mapping in a commercially available OCTA machine (RTVue XR Avanti™ with AngioVue™ and AngioAnalytics™ software, OptoVue, Freemont, USA). A density of >50% perfused vessels appears as bright red, dark blue represents no perfused vessels, and intermediate perfusion density is colour coded accordingly. Differences between the vessel perfusion density map of the superficial plexus in a 32-year-old healthy patient (A,B) and an age-matched subject with severe nonproliferative DR (C,D).
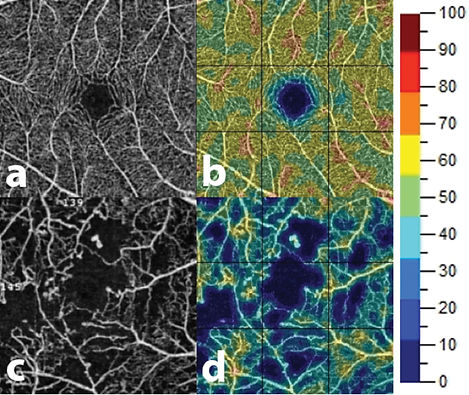
Agemy et al[14] were able to demonstrate a decrease in perfusion density in the superficial and deep retinal capillaries and choriocapillaris in all stages of DR when compared to healthy controls. Additionally, trend analysis showed a significant decrease in capillary perfusion density values as retinopathy progresses for most layers. The authors concluded that OCTA may offer an objective method for monitoring disease progression in diabetic retinopathy, acting as a potential tool for grading the disease in the future. Dimitrova et al[36] also reported that both superficial and deep retinal vessel density are decreased in the parafoveal area of diabetes patients without DR compared to healthy subjects. Ishibazawa et al[32] were able to calculate the neovessels’ area on OCTA of diabetes subjects and demonstrated a decrease in their values during follow up after the application of panretinal photocoagulation.
Limitations of OCTA
OCTA is associated with a few limitations that hinder its use in daily practice.
Artifacts – ie, additional or missing pieces of information or translation – are a common confounder to the accurate visualization and interpretation of images.[37] They can be caused by factors related to the eye (intrinsic characteristics or eye motion) or to the image (acquisition, processing, and display).[18] To avoid interpretation errors further to artifacts, OCTA should be accompanied by clinical evaluation, and OCTA images that are of poor quality (signal strength index ≤40) or display residual motion should be excluded from analysis.[38]
OCTA may also miss blood flow below a minimum threshold, as determined by the time between sequential OCT b-scans.[39] This may occur in microaneurysms or fibrotic choroidal neovascularization, for example.
As previously stated, OCTA is also limited to a relatively small field of view.
Conclusion
OCTA is a safe, rapid, and noninvasive procedure that allows 3-dimensional assessment of the chorioretinal layers in DR and has the potential to be employed in the screening and monitoring of patients with DR. Additional studies and clinical trials are needed to better elucidate how OCTA can be optimally incorporated into clinical practice and guide treatment decisions.
Dr. Oliveira is a Retina and Uveitis Specialist at the Instituto Panamericano da Visão, Goiânia, Brazil, and a Retinal Research Fellow at the Toronto Retina Institute, Toronto, Ontario. Dr. Berger is Assistant Professor, Department of Ophthalmology & Vision Sciences, University of Toronto, and Director of the Toronto Retina Institute. Dr. Chow is Assistant Professor, Department of Ophthalmology & Vision Sciences, University of Toronto, and Co-Director of the Toronto Retina Institute.
References:
-
International Diabetes Federation. IDF Diabetes Atlas, 8th Edition. 2017. Available at: www.diabetesatlas.org. Accessed on January 8, 2018
-
Diabetes Canada. Diabetes statistics in Canada. Available at: http://www.diabetes.ca/how-you-can-help/advocate/why-federal-leadership-is-essential/diabetes-statistics-in-canada#_ftn1. Accessed on August 3, 2017.
-
Canadian Diabetes Association. Clinical Practice Guidelines for the Prevention and Management of Diabetes in Canada. Can J Diabetes. 2013;37(Suppl 1):S8-S11.
-
Yau JW, Rogers SL, Kawasaki R, et al; Meta-Analysis for Eye Disease (META-EYE) Study Group. Global prevalence and major risk factors of diabetic retinopathy. Diabetes Care. 2012;35(3):556-564.
-
Flaxman SR, Bourne RRA, Resnikoff S, et al; Vision Loss Expert Group of the Global Burden of Disease Study. Global causes of blindness and distance vision impairment 1990-2020: a systematic review and meta-analysis. Lancet Glob Health. 2017; 5(12):e1221-e1234.
-
Zheng Y, He M, Congdon N. The worldwide epidemic of diabetic retinopathy. Indian J Ophthalmol. 2012;60(5):428-431.
-
Song SJ, Han K, Choi KS, et al; Task Force Team for Diabetes Fact Sheet of the Korean Diabetes Association. Trends in diabetic retinopathy and related medical practices among type 2 diabetes: results from the National Insurance Service Survey 2006-2013. J Diabetes Investig. 2017 Mar 10. [Epub ahead of print]
-
Sabanayagam C, Yip W, Ting DS, Tan G, Wong TY. Ten emerging trends in the epidemiology of diabetic retinopathy. Ophthalmic Epidemiol. 2016;23(4):209-222.
-
Mathur R, Bhaskaran K, Edwards E, et al. Population trends in the 10-year incidence and prevalence of diabetic retinopathy in the UK a cohort study in the Clinical Practice Research Datalink 2004-2014. BMJ Open. 2017;7(2):e014444.
-
Antonetti DA, Klein R, Gardner TW. Diabetic retinopathy. N Engl J Med. 2012;366(13):1227-39.
-
Wu L, Fernandez-Loaiza P, Sauma J, Hernandez-Bogantes E, Masis M. Classification of diabetic retinopathy and diabetic macular edema. World J Diabetes. 2013;4(6):290-294.
-
Hwang TS, Jia Y, Gao SS, et al. Optical coherence tomography angiography features of diabetic retinopathy. Retina. 2015;35(11):2371-2376.
-
Novotny HR, Alvis DL. A method of photographing fluorescence in circulating blood in the human retina. Circulation. 1961;24:82-86.
-
Agemy SA, Scripsema NK, Shah CM, et al. Retinal vascular perfusion density mapping using optical coherence tomography angiography in normals and diabetic retinopathy patients. Retina. 2015;35(11):2353-2363.
-
Ha SO, Kim DY, Sohn CH, Lim KS. Anaphylaxis caused by intravenous fluorescein: clinical characteristics and review of literature. Intern Emerg Med. 2014;9(3):325-330.
-
Su Z, Ye P, Teng Y, Zhang L, Shu X. Adverse reaction in patients with drug allergy history after simultaneous intravenous fundus fluorescein angiography and indocyanine green angiography. J Ocul Pharmacol Ther. 2012;28(4):410-413.
-
Lira RP, Oliveira CL, Marques MV, Silva AR, Pessoa Cde C. Adverse reactions of fluorescein angiography: a prospective study. Arq Bras Oftalmol. 2007;70(4):615-618.
-
Spaide RF, Fujimoto JG, Waheed NK. Image artifacts in optical coherence tomography angiography. Retina. 2015;35(11):2163-2180.
-
de Carlo TE, Romano A, Waheed NK, Duker JS. A review of optical coherence tomography angiography (OCTA). Int J Retina Vitreous. 2015;1:5.
-
De Oliveira PR, Berger AR, Chow DR. Optical coherence tomography angiography in chorioretinal disorders. Can J Ophthalmol. 2017;52(1):125-136.
-
Matsunaga DR, Yi JJ, De Koo LO, Ameri H, Puliafito CA, Kashani AH. Optical coherence tomography angiography of diabetic retinopathy in human subjects. Ophthalmic Surg Lasers Imaging Retina. 2015;46(8):796-805.
-
Ishibazawa A, Nagaoka T, Takahashi A, et al. Optical coherence tomography angiography in diabetic retinopathy: a prospective pilot study. Am J Ophthalmol. 2015;160(1):35-44.
-
Couturier A, Mane V, Bonnin S, et al. Capillary plexus anomalies in diabetic retinopathy on optical coherence tomography angiography. Retina. 2015;35(11):2384-2391.
-
Kim DY, Fingler J, Zawadzki RJ, et al. Noninvasive imaging of the foveal avascular zone with high-speed, phase-variance optical coherence tomography. Invest Ophthalmol Vis Sci. 2012;53(1):85-92.
-
Conrath J, Giorgi R, Raccah D, Ridings B. Foveal avascular zone in diabetic retinopathy: quantitative vs qualitative assessment. Eye (Lond). 2005;19(3):322-326.
-
Takase N, Nozaki M, Kato A, Ozeki H, Yoshida M, Ogura Y. Enlargement of foveal avascular zone in diabetic eyes evaluated by en face optical coherence tomography angiography. Retina. 2015;35(11): 2377-2383.
-
Di G, Weihong Y, Xiao Z, et al. A morphological study of the foveal avascular zone in patients with diabetes mellitus using optical coherence tomography angiography. Graefes Arch Clin Exp Ophthalmol. 2016;254(5):873-879.
-
Freiberg FJ, Pfau M, Wons J, Wirth MA, Becker MD, Michels S. Optical coherence tomography angiography of the foveal avascular zone in diabetic retinopathy. Graefes Arch Clin Exp Ophthalmol. 2016;254(6): 1051-1058.
-
de Carlo TE, Chin AT, Bonini Filho MA, et al. Detection of microvascular changes in eyes of patients with diabetes but not clinical diabetic retinopathy using optical coherence tomography angiography. Retina. 2015;35(11):2364-2370.
-
Lee CS, Lee AY, Sim DA, et al. Reevaluating the definition of intraretinal microvascular abnormalities and neovascularization elsewhere in diabetic retinopathy using optical coherence tomography and fluorescein angiography. Am J Ophthalmol. 2015;159(1):101-110.
-
de Carlo TE, Bonini Filho MA, Baumal CR, et al. Evaluation of preretinal neovascularization in proliferative diabetic retinopathy using optical coherence tomography angiography. Ophthalmic Surg Lasers Imaging Retina. 2016;47(2):115-159.
-
Ishibazawa A, Nagaoka T, Yokota H, et al. Characteristics of retinal neovascularization in proliferative diabetic retinopathy imaged by optical coherence tomography angiography. Invest Ophthalmol Vis Sci. 2016;57(14):6247-6255.
-
Savastano MC, Federici M, Falsini B, Caporossi A, Minnella AM. Detecting papillary neovascularization in proliferative diabetic retinopathy using optical coherence tomography angiography. Acta Ophthalmol. 2016 Aug 6. [Epub ahead of print]
-
de Carlo TE, Chin AT, Joseph T, et al. Distinguishing diabetic macular edema from capillary nonperfusion using optical coherence tomography angiography. Ophthalmic Surg Lasers Imaging Retina. 2016;47(2):108-114.
-
Moisseiev E, Loewenstein A. Diabetic macular edema: emerging strategies and treatment algorithms. Dev Ophthalmol. 2017;60:165-174.
-
Dimitrova G, Chihara E, Takahashi H, Amano H, Okazaki K. Quantitative retinal optical coherence tomography angiography in patients with diabetes without diabetic retinopathy. Invest Ophthalmol Vis Sci. 2017;58(1):190-196.
-
Ghasemi Falavarjani K, Al-Sheikh M, Akil H, Sadda SR. Image artefacts in swept-source optical coherence tomography angiography. Br J Ophthalmol. 2017;101(5):564-568.
-
Chalam KV, Sambhav K. Optical coherence tomography angiography in retinal diseases. J Ophthalmic Vis Res. 2016;11(1):84-92.
-
de Carlo TE, Romano A, Waheed NK, Duker JS. A review of optical coherence tomography angiography (OCTA). Int J Retina Vitreous. 2015;1:5.
Disclosure: Drs. Oliveira and Berger stated that they have no disclosures to report in association with the contents of this issue. Dr. Chow is a consultant for Alcon, Bayer, Dutch Ophthalmic Research Center, Syngergetics, and Katalyst, and a lecturer for Allergan, Dutch Ophthalmic Research Center, and Optovue.
Ophthalmology Rounds is made possible through educational support from