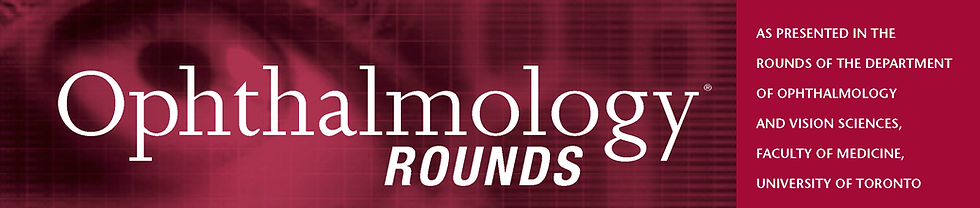
Update on Age-Related Macular Degeneration – Part 1: Current and Future Trends in Diagnosis
By DAVID TRI TA KIM, MD, FRCSC, and ALAN RICHARD BERGER, MD, FRCSC
Age-related macular degeneration (AMD) is a leading cause of vision loss in patients aged ≥50 years and especially those living in the developed world. The number of patients with this disease is expected to rise as the population ages. This edition of Ophthalmology Rounds will review the current diagnostic options for patients at elevated risk of or who are suspected of having AMD, and will also explore future trends. The next issue will present the available management strategies for dry and wet AMD.
Age-related macular degeneration (AMD) is among the most common causes of blindness in patients in the developed world and the fourth most common cause worldwide.¹⁻⁵ The pooled global AMD prevalence is 8.7%; however, it was found to be higher among Europeans than Asians or Africans.⁵ As its name implies, increased age is the principal risk factor for AMD. A European systematic review and meta-analysis estimated that the prevalence of early or intermediate AMD was 25.3% and of any late AMD was 2.4% among patients aged ≥60 years,² and a global meta-analysis determined that >14% of blindness was secondary to AMD in patients aged ≥50 years in high-income subregions.⁴ Population aging was projected to increase the prevalence of AMD worldwide by 46% worldwide in 2040 from 2020 estimates (288 million from 196 million cases).⁵
Clinical Features
Drusen are the hallmark of AMD. They present as yellowish deposits in the retinal pigment epithelium (RPE) or in the Bruch’s membrane. Drusen can be described according to their size: small (<64 µm in diameter), intermediate
(64–124 µm), or large (≥125 µm). They can also be described by their boundaries: hard, soft, or confluent. The presence of a few small hard drusen does not seem to be a risk factor for symptomatic AMD as they are commonly found on histopathology specimens in patients aged ≥50 years.⁶
Reticular pseudodrusen, also known as subretinal drusenoid deposits or reticular drusen, appear as a network of individual yellowish deposits (Figure 1). Regression of these lesions has been shown to be associated with outer retinal atrophy and decrease of the underlying choroidal thickness.⁷ The presence of reticular pseudodrusen is an independent predictive factor for progression to late AMD, as per the classification developed by the Age-related Eye Disease Study (AREDS) group (Table 1),⁸⁻⁹ although the magnitude is much less than with soft drusen; as demonstrated in a prospective case-control study, the odds ratio of developing advanced AMD in patients with reticular pseudodrusen was 2.6 compared with 16.6 for soft drusen.¹⁰
Figure 1: Subretinal drusenoid deposits.

This image was originally published in the Retina Image Bank® website. Author: Gerardo Garcia-Aguirre, MD. Subretinal drusenoid deposits. Retina Image Bank. 2018; Image Number 28339. © the American Society of Retina Specialists.
Table 1: Classification of AMD developed by the AREDS group⁸⁻⁹
Vitelliform lesions are seen on fundus examination as round lesions with an egg yolk-like appearance usually centred on the fovea, representing an accumulation of yellowish deposits in the subretinal space. These lesions are uncommon in eyes with typical dry AMD. They are thought to occur due to impaired phagocytosis of photoreceptor outer segments, although it is unknown whether they form as a result of the pathogenesis of AMD or a second disease process. Vitelliform lesions usually occur as a single lesion in the fovea but can also be found in multiple noncentral macular areas.¹¹ Regression of vitelliform lesions is associated with atrophy of the ellipsoid and RPE. They can be infrequently associated with the development of choroidal neovascular membranes.
Other clinical findings in AMD include focal RPE hyperpigmentation and geographic and nongeographic atrophy. Geographic atrophy (GA) is an area of circumscribed or patchy atrophy of the RPE, usually found in the central or paracentral macular area. Photoreceptors over areas of GA usually degenerate, leading to vision loss. Regression of large drusen is associated with the development of GA, and the total macular area occupied by drusen is a risk factor for GA.¹² The sequence of events leading to GA has been proposed as starting with drusen, followed by the appearance of focal hyperpigmentation areas, regression of drusen, appearance of hypopigmentation, and finally, GA.¹³ This condition can also occur following the collapse of a pigment epithelial detachment (PED), particularly drusenoid PEDs.¹⁴ GA can also occur in areas without drusen, as well as appear around the fovea; in the latter case, GA spreads in a horseshoe pattern, eventually encircling and affecting the fovea.
In addition to their classification system, the AREDS group also created a simplified grading scale to classify AMD severity based on the presence or absence of the following retinal abnormalities: ≥1 large (>125 µm diameter) drusen (1 point); pigment abnormalities (1 point); for patients with no large drusen, bilateral intermediate
(64–124 µm) drusen (1 point); and neovascular AMD (2 points).¹⁵ These risk factors are summed for both eyes to estimate the 5- and 10-year risk of developing advanced AMD (Table 2).
Table 2: Five- and 10-year risks of development of late AMD based on a simplified grading scale
developed by the AREDS group¹⁵
Choroidal neovascularization (CNV) is the hallmark of neovascular (“wet”) AMD. CNV is one or more areas of vascular ingrowth from the choroid through the Bruch’s membrane and into the sub-RPE, subretinal, or intraretinal space. CNV usually causes fluid leakage into these spaces with resultant visual symptoms. On fundus examination, the CNV can sometimes be seen as a gray-green elevation of tissue deep in the retina. CNV is often not visible and its presence is deduced from the visualization of blood, lipid, subretinal fluid, or PED (Figure 2). These clinical findings can be subtle therefore a thorough clinical examination is imperative.
Figure 2: 80-year-old patient, PED / CNVM.

This image was originally published in the Retina Image Bank® website. Author: David Callanan, MD. Title: PED / CNVM. Retina Image Bank. 2014; Image Number 21443. © the American Society of Retina Specialists.
PEDs occur when the thickened inner aspect of the Bruch’s membrane and the RPE detach from the rest of the membrane. PEDs can be drusenoid, serous, fibrovascular, or hemorrhagic. Drusenoid PEDs are not associated with CNV; however, in the AREDS, eyes with drusenoid PEDs had a 42% chance of progressing to advanced AMD at 5 years, with an even split between foveal GA and CNV.¹²⁻¹⁴ Fibrovascular and hemorrhagic PEDs are associated with CNV, as may serous PEDs. Clinical signs suggesting CNV associated with a PED include subretinal fluid, lipid, blood, and chorioretinal folds.¹⁶ Intravenous fluorescein angiography (IVFA) or optical coherence tomography angiography (OCT-A) can differentiate the types of PED and whether CNV is present.
Retinal angiomatous proliferation is a form of CNV that is believed to arise from within the retina. The retinal vessels are seen to be anastomosing with the neovascular membrane. It is uncertain whether these vessels develop prior to or after CNV. On OCT, the most common features are a serous PED with overlying CME.
Massive subretinal hemorrhage is an uncommon complication of wet AMD but has devastating results on the visual prognosis. Breakthrough bleeding with vitreous hemorrhage can occur if blood passes through the retina into the vitreous cavity.
RPE tears, also known as RPE rips, are often associated with CNV, especially when a PED is present. They can occur either spontaneously, after photodynamic therapy or after antiangiogenesis treatment.¹⁷⁻¹⁸ The PED is thought to stretch the RPE, eventually leading to a tear. The sheet of RPE cells then scrolls on itself, leaving an area of bare RPE. The visual prognosis is poor when the RPE tear extends under the fovea.
Disciform scars are the end stage of CNV and are characterized by sub-RPE or subretinal fibrosis. Central vision is usually severely compromised when the scar extends under the foveal centre. New areas of neovascularization can appear along the edge and affect previously unaffected areas of the retina (Figure 3).
Figure 3: Disciform scar with active area of neovascular AMD inferotemporally.
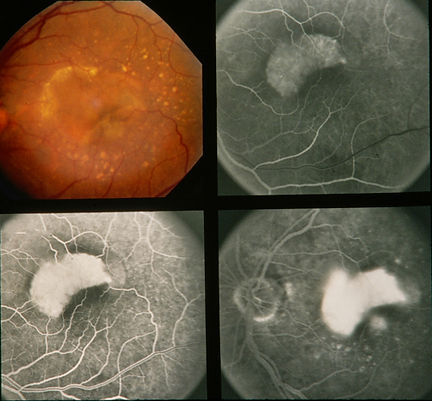
This image was originally published in the Retina Image Bank® website. Author: Mitzy E Torres Soriano, MD. Title: Active Neovascular AMD With Disciform Scar. Retina Image Bank. 2015; Image Number 24986. © the American Society of Retina Specialists.
Home Monitoring
Amsler grid
The Amsler grid is a screening test card with grid lines and a central dot for fixation. It is offered to patients so that they can self-monitor for new visual symptoms that might suggest progression of their AMD. Patients are told to check each eye individually for new areas of metamorphopsia, scotoma, or other changes that would prompt an evaluation with their ophthalmologist. In a meta-analysis, the Amsler grid was found to have a sensitivity of 64%–87% and specificity of 91%–99% to detect CNV; however, the authors note that many studies used different and possibly suboptimal reference tests that could affect the validity of the meta-analysis.¹⁹ Another publication showed that many patients are not properly educated on how to perform and interpret their Amsler grid test.²⁰
Diagnostic Tests
IVFA
AMD is associated with many different findings on IVFA. Hyperfluorescent lesions can be associated with drusen, RPE atrophy, RPE tears, serous PEDs, CNV, subretinal fibrosis, and laser scars. Hypofluorescent lesions can represent hemorrhages at any level, lipid, or pigment proliferation.
CNV can be described as classic or occult. Classic lesions consist of a discrete, well-demarcated focal area of hyperfluorescence that can be discerned in the early phases of the angiogram. Through the middle and late phases, hyperfluorescence increases in intensity and extends past the boundaries of the area identified in the early phase. Occult CNV actually refers to 2 patterns of hyperfluorescence on IVFA. The first pattern is called a fibrovascular PED and presents with an irregular elevation of the RPE stippled with hyperfluorescent dots. The boundaries may or may not be sharply outlined. The second pattern is a late leakage of an undetermined source. There is no clearly identifiable classic or fibrovascular PED pattern lesion in the early and middle phases of the angiogram to account for the area of leakage in the late phase. The boundaries of this pattern cannot usually be determined. This classification of CNV was more commonly used in the past as it had an impact on the choice of therapeutic management. In the antiangiogenesis era, the classification is uncommonly used in clinical practice. Classic CNVs are more likely to progress and have more severe visual loss.²¹
The different types of PEDs (fibrovascular, serous, hemorrhagic, and drusenoid) can be differentiated on IVFA. Fibrovascular PEDs show a stippled hyperfluorescence by the middle phase with pooling of the dye in the late phase. Serous PEDs show uniform bright hyperfluorescence in the early phase with minimal or absent leakage at the border in the late phase. Hemorrhagic PEDs will block the choroidal fluorescence. Drusenoid PEDs occur in areas of large confluent drusen and fluoresce faintly.
Disciform scars frequently hyperfluoresce due to leaking and staining.
Fundus autofluorescence (FAF)
Drusen by themselves do not correlate with any significant findings on FAF; however, GA appears as sharply defined areas of hypoautofluorescence due to the loss of RPE and corresponding loss of lipofuscin accumulation. Hyperautofluorescence in the junctional area, which borders on nonatrophic tissue, is associated with more rapid GA progression.²² The use of FAF is important in monitoring for progression of dry AMD and GA.
OCT
Since its introduction in 1991, OCT has revolutionized the diagnosis of AMD by producing a noninvasive, high-resolution, cross-sectional image of the retina.²³ The first generation of OCT devices used in the clinic were time-domain OCTs and used a broadband light source and a moving reference mirror to acquire images. Spectral-domain (SD) OCT was the next technological advance; it removed the moving reference mirror, allowing for faster image acquisition. SD-OCT also improved axial resolution. The latest iteration of OCT technology, swept-source (SS) OCT, uses a narrow-band light source, which allows deeper penetration of tissue and better resolution of structures such as the choroid.²⁴⁻²⁵
Drusen can be seen on OCT as slight elevations of the RPE or as convex-shaped deposits with low, medium, or high internal reflectivity (Figure 4).²⁶ Drusen with medium reflectivity are the most common; however, eyes with low or high internal reflectivity are more likely to have areas of RPE atrophy, which has already been established as a risk factor for progression to advance AMD.²⁷ There can be thinning of the photoreceptor layer and alterations of the ellipsoid zone.
Figure 4: OCT demonstrating the presence of multiple drusen as well as hyperreflective foci can be found just anterior to drusen.
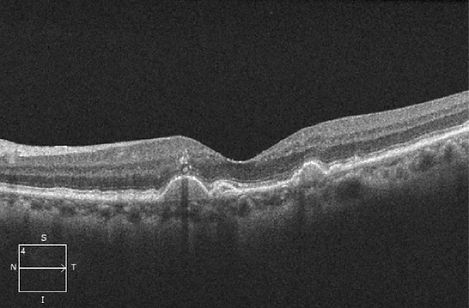
Moderate to intense hyperreflective foci can be found just anterior to drusen (Figure 4) but also in the more anterior retinal layers. They correspond to the foci of hyperpigmentation on clinical examination. An ancillary study of SD-OCT findings in AREDS2 patients found that eyes with drusen and hyperreflective foci were more likely to progress to GA within 2 years by a factor of 4.7.28 The magnitude of this risk factor was increased by the number of hyperreflective foci as well as the more anterior location.²⁸
In areas of GA, the outer nuclear layer, external limiting membrane, and ellipsoid zone are absent with the RPE and Bruch’s membrane either absent or markedly attenuated. The choroid seems more hyperreflective due to the window transmission defect (Figure 5).
Figure 5: OCT demonstrating subfoveal geographic atrophy with absence of the outer retinal nuclear layer, external limiting membrane, and ellipsoid zone. The choroid under the area of GA is more hyperreflective due to the window defect.

An outer retinal tubulation (ORT) is an OCT finding in the outer nuclear layer that presents as a thick reflective line surrounding a tubular hyporeflective cavity. ORTs differentiate themselves from cystoid anomalies, which do not have this thick reflective line surrounding the cavity. ORTs are thought to represent degenerated red-green cones and Müller cells, with the hyperreflective line being the mitochondria.²⁹⁻³⁰ It is important to identify ORTs as these remain chronic changes and are unlikely to respond to anti-vascular endothelial growth factor (VEGF) injections.
The advent of OCT has allowed clinicians to easily see intraretinal (Figure 6), subretinal (Figure 7), and sub-RPE fluid, and to follow subtle changes serially and objectively. Lipid exudation can be seen appearing as hyperreflective dots in the outer retina. Serous PEDs are seen as dome-shaped elevations of the RPE overlying a homogenously hyporeflective space with the Bruch’s membrane visible below (Figures 7, 8). Fibrovascular PEDs appear to be filled with solid layers of medium reflective material separated by hyporeflective clefts (Figure 9). Hemorrhagic PEDs are dome-shaped, similar to serous PEDs; however, the sub-RPE blood appears hyperreflective, attenuating the visibility of deeper structures.
Figure 6: OCT demonstrating multiple drusen and intraretinal fluid.

Figure 7: OCT demonstrating a serous PED, characterized by homogenously hyporeflective space, with subretinal fluid.
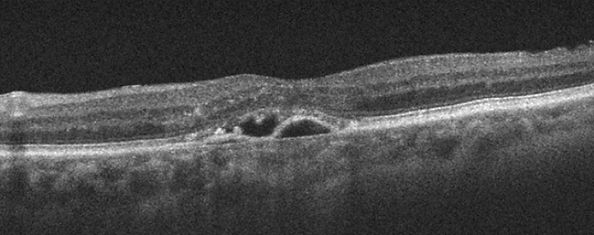
Figure 8: OCT demonstrating serous PED, again characterized by homogenously hyporeflective space.
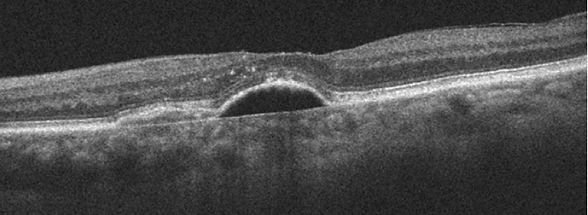
Figure 9: OCT demonstrating a fibrovascular PED, characterized by solid layers of medium reflective material (white arrow) separated by hyporeflective clefts (black arrow), with subretinal fluid.
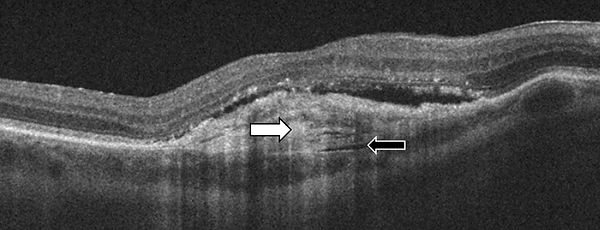
OCT-A
OCT-A is a new rapid, noninvasive, flow-based test that provides images of the retinal and choroidal vasculature. OCT-A can be performed using SD or SS technology. Instead of having to use intravenous dyes, OCT-A generates vascular maps using motion contrast by taking sequential OCT B-scans in the same cross-sectional location. Numerous cross-sectional locations are scanned to give a volumetric or 3-dimensional vascular map. Automated segmentation generates en face angiograms in 2 dimensions, allowing the clinician to study different levels of the retina; eg, superficial retina or choriocapillaris. The data can also be viewed in the cross-sectional B scan with colour-coded flow data, thereby allowing for the identification of areas with blood flow (Figure 10).
Figure 10: 71-year-old man presenting with new onset wet AMD.
Top: Fundus photograph showing a choroidal neovascular membrane (CNVM) with subretinal fluid and subretinal hemorrhage.
Bottom left: En face OCT-A of the CNVM.
Bottom right: Cross-sectional view of the CNVM with the blood flow coded in red. The automated segmentation was done in the normally avascular outer retina, the limits of which are delineated by the dashed purple lines generating the en face image seen on the left.

Advantages of OCT-A over IVFA include faster acquisition times (seconds vs minutes), noninvasive nature, more precise depth localization of findings, and possible better resolution.³¹ Disadvantages include inability to detect leakage, relatively small field of view in current iterations, and inability to differentiate low flow from lack of flow such as with microaneurysms in diabetic retinopathy.³¹
For dry AMD, OCT-A can be used to exclude CNV as well as to track the progression of geographic atrophy as it has been shown to reliably quantify the extent of GA.³²
In wet AMD, OCT-A can be used to identify asymptomatic CNV, which is CNV without any signs of exudation on examination or structural OCT. Asymptomatic CNV has been shown to have a greater risk of exudation than eyes without asymptomatic CNV (21% vs 4% by 12 months).³³ These eyes may benefit from closer follow-up. In these cases, OCT-A can also be used to serially monitor for CNV growth although there is currently no evidence from clinical trials demonstrating that serial OCT-A is of any benefit in the management of active CNV. An additional use of OCT-A is in cases of suspected CNV with equivocal conventional IVFA findings, such as where differentiation of the collapse of an acquired vitelliform lesion from active CNV proves difficult on IVFA as the subretinal vitelliform material stains intensely.³⁴
Summary
Diagnostic testing options for both dry and wet AMD have greatly advanced over the years and continue to improve. SS-OCT allows for better resolution, wider view, and greater resolution of deeper tissue. OCT-A allows for rapid, noninvasive evaluation of the retinal and choroidal vasculature although it has not completely replaced the role of IVFA.
References
-
Friedman DS, O’Colmain BJ, Muñoz B, et al; Eye Diseases Prevalence Research Group. Prevalence of age-related macular degeneration in the United States. Arch Ophthalmol. 2004;122(4):564-572.
-
Li JQ, Welchowski T, Schmid M, Mauschitz MM, Holz FG, Finger RP. Prevalence and incidence of age-related macular degeneration in Europe: a systematic review and meta-analysis. Br J Ophthalmol. 2020;104(8):1077-1084.
-
Maberley DAL, Hollands H, Chuo J, et al. The prevalence of low vision and blindness in Canada. Eye. 2006;20(3):341-346.
-
Flaxman SR, Bourne RRA, Resnikoff S, et al; Vision Loss Expert Group of the Global Burden of Disease Study. Global causes of blindness and distance vision impairment 1990–2020: a systematic review and meta-analysis. Lancet Glob Health. 2017;5(12):e1221-1234.
-
Jonas JB, Gemmy Cheung CM, Panda-Jonas S. Updates on the epidemiology of age-related macular degeneration. Asia-Pac J Ophthalmol. 2017;6(6):493-497.
-
Coffey AJ, Brownstein S. The prevalence of macular drusen in postmortem eyes. Am J Ophthalmol. 1986;102(2):164-171.
-
Spaide RF. Outer retinal atrophy after regression of subretinal drusenoid deposits as a newly recognized form of late age-related macular degeneration. Retina. 2013;33(9):1800-1808.
-
Age-Related Eye Disease Study Research Group, 2001. A randomized, placebo-controlled, clinical trial of high-dose supplementation with vitamins C and E, beta carotene, and zinc for age-related macular degeneration and vision loss: AREDS report no. 8. Arch Ophthalmol. 2001;119(10):1417-1436.
-
Bressler NM, Bressler SB. Age-related macular degeneration: non-neovascular early AMD, intermediate AMD, and geographic atrophy. In Ryan SJ, Schachat AP, Wilkinson CP, Hinton DR, Wiedemann P (eds.). Retina, Fifth Edition. Elsevier Health Sciences. 2013. Beijing, China. Page 1160.
-
Zweifel SA, Imamura Y, Spaide TC, Fujiwara T, Spaide RF. Prevalence and significance of subretinal drusenoid deposits (reticular pseudodrusen) in age-related macular degeneration. Ophthalmology. 2010;117(9):1775-1781.
-
Freund KB, Laud K, Lima LH, Spaide RF, Zweifel S, Yannuzzi LA. Acquired vitelliform lesions: correlation of clinical findings and multiple imaging analyses. Retina. 2011;31(1):13-25.
-
Complications of Age-related Macular Degeneration Prevention Trial (CAPT) Research Group. Risk factors for choroidal neovascularization and geographic atrophy in the complications of age-related macular degeneration prevention trial. Ophthalmology. 2008;115(9):1474-1479, 1479.e1-6.
-
Brader HS, Ying G, Martin ER, Maguire MG. Characteristics of incident geographic atrophy in the Complications of Age-related Macular Degeneration Prevention Trial. Ophthalmology. 2013;120(9):1871-1879.
-
Cukras C, Agrón E, Klein ML, et al; Age-Related Eye Disease Study Research Group. Natural history of drusenoid pigment epithelial detachment in age-related macular degeneration: AREDS Report Number 28. Ophthalmology. 2010;117(3):489-499.
-
Ferris FL, Davis MD, Clemons TE, et al; Age-Related Eye Disease Study Research Group. A simplified severity scale for age-related macular degeneration: AREDS Report No. 18. Arch Ophthalmol. 2005;123(11):1570-1574.
-
Bressler NM, Bressler SB, Fine SL. Age-related macular degeneration. Surv Ophthalmol. 1988;32(6):375-413.
-
Gelisken F, Inhoffen W, Partsch M, Schneider U, Kreissig I. Retinal pigment epithelial tear after photodynamic therapy for choroidal neovascularization. Am J Ophthalmol. 2001;131(4):518-520.
-
Meyer CH, Mennel S, Schmidt JC, Kroll P. Acute retinal pigment epithelial tear following intravitreal bevacizumab (Avastin) injection for occult choroidal neovascularisation secondary to age related macular degeneration. Br J Ophthalmol. 2006;90(9):1207-1208.
-
Faes L, Bodmer NS, Bachmann LM, Thiel MA, Schmid MK. Diagnostic accuracy of the Amsler grid and the preferential hyperacuity perimetry in the screening of patients with age-related macular degeneration: systematic review and meta-analysis. Eye (Lond). 2014;28(7):788-796.
-
Fine AM, Elman MJ, Ebert JE, Prestia PA, Starr JS, Fine SL. Earliest symptoms caused by neovascular membranes in the macula. Arch Ophthalmol. 1986;104(4):513-514.
-
Gregori G, Wang F, Rosenfeld PJ, Yehoshua Z, Gregori NZ, Lujan BJ, et al. Spectral domain optical coherence tomography imaging of drusen in nonexudative age-related macular degeneration. Ophthalmology. 2011; 118(7):1373-1379.
-
Schmitz-Valckenberg S, Fleckenstein M, Scholl HPN, Holz FG. Fundus autofluorescence and progression of age-related macular degeneration. Surv Ophthalmol. 2009;54(1):96-117.
-
Huang D, Swanson EA, Lin CP, et al. Optical coherence tomography. Science. 1991;254(5035):1178-1181.
-
Gabriele ML, Wollstein G, Ishikawa H, et al. Optical Coherence Tomography: History, current status, and laboratory work. Invest Ophthalmol Vis Sci. 2011;52(5):2425-2436.
-
Lavinsky F, Lavinsky D. Novel perspectives on swept-source optical coherence tomography. Int J Retina Vitreous. 2016;2:25.
-
Khanifar AA, Koreishi AF, Izatt JA, Toth CA. Drusen ultrastructure imaging with spectral domain optical coherence tomography in age-related macular degeneration. Ophthalmology. 2008;115(11):1883-1890.
-
Leuschen JN, Schuman SG, Winter KP, et al. Spectral-Domain Optical Coherence Tomography Characteristics of Intermediate Age-Related Macular Degeneration. Ophthalmology. 2013;120(1):140-150.
-
Christenbury JG, Folgar FA, O’Connell R, Chiu SJ, Farsiu S, Toth CA. Progression of intermediate age-related macular degeneration with proliferation and inner retinal migration of hyperreflective foci. Ophthalmology. 2013;120(5):1038-1045.
-
Curcio CA, Medeiros NE, Millican CL. Photoreceptor loss in age-related macular degeneration. Invest Ophthalmol Vis Sci. 1996;37(7):1236-1249.
-
Schaal KB, Freund KB, Litts KM, Zhang Y, Messinger JD, Curcio CA. Outer retinal tubulation in advanced age-related macular degeneration: optical coherence tomographic findings correspond to histology. Retina. 2015; 35(7):1339-1350.
-
Schneider EW, Fowler SC. Optical coherence tomography angiography in the management of age-related macular degeneration. Curr Opin Ophthalmol. 2018;29(3):217-225.
-
Corbelli E, Sacconi R, Rabiolo A, et al. Optical coherence tomography angiography in the evaluation of geographic atrophy area extension. Invest Ophthalmol Vis Sci. 2017;58(12):5201-5208.
-
de Oliveira Dias JR, Zhang Q, Garcia JMB, et al. Natural history of subclinical neovascularization in nonexudative age-related macular degeneration using swept-source OCT angiography. Ophthalmology. 2018;125(2):255-266.
-
Joshi KM, Nesper PL, Fawzi AA, Mirza RG. Optical coherence tomography angiography in adult-onset foveomacular vitelliform dystrophy. Retina. 2018;38(3):600-605.
Dr. Ta Kim is a vitreoretinal surgery fellow at St. Michael’s Hospital, Toronto, Ontario. Dr. Berger is an academic vitreoretinal surgeon at St. Michael’s Hospital, and a Vice Chairman of the University of Toronto Department of Ophthalmology and is co-director of the Toronto Retina Institute.
Disclosure Statement: Dr. Ta Kim stated that he has no disclosures to report in association with the contents of this issue. Dr. Berger receives honoraria and is a consultant for Bayer Canada, Novartis Pharmaceuticals Canada, Allergan, and Roche Canada.
Ophthalmology Rounds is made possible through educational funding
from the following industry co-sponsors: